Improvements in National Data Buoy Center Measurements
David B. GilhousenNational Data Buoy Center
Stennis Space Center, MS 39529-6000
ABSTRACT
Several significant improvements in moored buoy wind and wave measurements were made by the National Data Buoy Center (NDBC) in the 1980's. These improvements, which will be documented as best as possible, will be important to anyone using the data to analyze long-term climatic trends. A recent technique to estimate swell height and period from spectral wave measurements will be presented. This technique could be applied on historical data to enable better merging of our wave records with ship observations, or to improve climatic summaries.
Several expansion measurements may be of interest to marine climatologists. Relative humidity sensors have improved to the point where they can be expected to last almost a year on a moored buoy. This measurement, which is archived as dew point, has been added to many Coastal-Marine Automated Network (C-MAN) and a few buoy stations in the 1990's. The National Aeronautics and Space Administration's (NASA) Tropical Rainfall Measuring Mission has funded NDBC for rainfall measurements from several buoys. The measurements to date have been made with an optical rain gauge, were of poor quality, and were not archived. A siphon rain gauge, in use with some success in the Tropical Ocean and Global Atmosphere (TOGA) TAO array, will be installed on these buoys this year.
Drifting buoy measurements of sea surface temperature have been accurate since the 1970's. Degraded sea level pressure measurements were greatly reduced in the period 1987 to 1989 as better monitoring and feedback methods were developed. The oceanographic community through the Global Ocean Observing System (GOOS), not NDBC, is now the driving force behind almost all of the world's drifting buoys. Most drifting buoys now in use are Lagrangian, which was not the case in the 1980's, and typically make sea level pressure and sea surface temperature measurements.
1. INTRODUCTION
Observations reported by NDBC moored buoy and C-MAN stations are one of the few sources of long time-series measurements in the marine environment. Several changes made through the years, either to reduce operational cost or improve data quality, have impacted the climatic continuity of the record. This paper will address these changes, along with those in drifting buoys, and provide some insight into the future directions of automated marine measurements.
2. WINDS
Well over 99 percent of NDBC's historical wind measurements have been taken by propellor anemometers. In the 1970's and early 1980's, Bendix aerovanes were used; since the mid-1980's R.M. Young Model 05103 has been the standard wind sensor. All wind sensors are calibrated before deployments to ensure that the reported speed lies within 1 m/s of the wind tunnel standard. From 1978 to 1982, a few buoy stations used vortex shedding anemometers. Though these no-moving-part anemometers performed well in the wind tunnel, occasionally snowfall or heavy precipitation would obstruct the orifice and lower the reported winds. Once NDBC discovered the problem, they removed data that were known to be degraded from the archives, and limited the deployments of these sensors until they were phased out. The impact on the historical record, say in terms of artificial lowering of monthly mean speeds, is negligible.
Two aspects of our wind measurements would impact climatic continuity. First, buoy hull types, and therefore anemometer heights, have changed through the years at most moored buoy stations. The 10- and 12-m discus hulls have anemometers at the 10-m height, while 6-m Navy Oceanographic and Meteorological Automatic Device (NOMAD) and 3-m discus hulls have anemometer heights of 5-m. Second, the older General Service Buoy Payloads (GSBP), or onboard computer systems, computed a vector average of wind speed, while more recent payloads compute a scalar average. Vector averaging results in speeds that are several percent lower when winds exceed 10 m/s (Gilhousen, 1987).
3. WAVES
An improvement in a processing method in June 1984 caused a significant data continuity problem at many stations. The implementation of a variable, or sea-state-dependent, noise correction caused an increase in low frequency, or swell energy. This amplification is most apparent when swells are small or mixed with wind-waves. In these situations, the overall significant wave height increases, and the dominant wave period can increase. Because the noise corrections decrease rapidly at higher frequencies, negligible changes occur in wind-wave energy above 0.12 Hz. A complete explanation of the technique and rationale is given in Earle, Steele, and Hsu (1984). Figure 1, taken from that paper, shows a typical difference in the magnitude of the low frequency spectral peak. Earle (1999) and Anctil (1993) have compared NDBC wave heights and periods with those from other wave measurement systems. Ninety-five percent of significant wave heights agree within 0.2 m, and 95 percent of dominant wave periods agree within 0.7 s. These excellent results would not have been possible without the variable noise corrections.
Because the variable noise correction method is based on the raw, uncorrected spectral densities at 0.01 and 0.02 Hz, and the uncorrected values are unavailable except for recent years, pre-1984 data cannot be adjusted. Unfortunately, NDBC did not have the computer capability in the 1970's and early 1980's to retain the uncorrected values. Because the spectrum above 0.12 Hz is unaffected, climatic data continuity is probably not affected in areas that have infrequent swells. This is certainly true of the Great Lakes and quite possibly the Atlantic and Gulf of Mexico. On the other hand, pre-1984 data for NDBC systems in the Pacific is clearly different than measurements taken since then.
Several other, more minor, concerns exist with continuity of wave reports. First, large 12-m discus buoys filter out small wind waves, say at frequencies above approximately 0.30 Hz. Fortunately, these monster buoys have been used at very few buoy stations. NDBC's Data Availability Summary gives the locations and times. Second, minor location differences near shore can mean sizeable changes in water depth that can limit wave height. Water depths are available on the National Oceanic and Atmospheric Administration (NOAA) Marine Environmental Buoy Data Web Discs from the National Oceanographic Data Center (NODC).
Finally, NDBC has developed a method to produce an estimate of swell height, swell period, wind wave height, and wind wave period, given the wave spectrum and the wind speed (Hervey, 1999). This method could be applied to historical data to produce swell climatologies or to facilitate comparison with ship observations.
4. AIR TEMPERATURE, WATER TEMPERATURE, AND SEA LEVEL PRESSURE
The same sensor types, often by the same manufacturer, have been used for these measurements. Other than differences due to sensor height, no climatic data continuity issues should exist. Again, sensor heights are tied to the hull type which are given in the NDBC Data Availability Summary.
5. DRIFTING BUOYS
Degraded sea level pressure measurements from drifting buoys often wreaked havoc with operational analyses and caused data management problems at archive centers in the mid-1980's. Compared with numerical weather prediction first guess fields for a month in 1987, 10 percent of NDBC drifting buoys had either a 1 hPa bias or a standard deviation of differences greater than 4 hPa. Thirty-three percent of the non-NDBC drifting buoys exhibited these characteristics. Occasionally, misleading air or water temperatures were transmitted from drifting buoys that had gone aground or had not yet been deployed. NDBC pioneered methods of quality controlling drifting buoy data and operationally implemented them on NDBC drifting buoys in November 1987 (Gilhousen, 1988). The World Meteorological Organization's (WMO) Data Buoy Cooperative Panel Technical Coordinator adopted many of these methods on worldwide buoys during 1988. These methods resulted in lowering the percent of degraded pressure measurements to 10 percent for all drifting buoys by late 1988.
A small percentage of drifting buoys reported wind speed, even fewer reported wind direction. Field evaluations of several NDBC wind-measuring drifting buoys are contained in Gilhousen (1993). Meteo France has also successfully obtained wind measurements from a drifting buoy (Daniault et al., 1984).
NDBC's last reporting drifting buoy ceased transmitting in July 1999. The oceanographic community through the GOOS, not NDBC, is now the driving force behind almost all of the world's drifting buoys. The Atlantic Oceanographic and Meteorological Laboratory's Global Drifter Center now coordinates worldwide deployment and archiving of drifting buoy data. Most drifting buoys used in the last 4 years are smaller, Lagrangian buoys called surface velocity profilers (SVP). Sea level pressure and sea surface temperature are routinely reported. For information on these drifting buoys as well as international drifting and moored buoy programs, consult the WMO's Data Buoy Cooperation Panel's Annual Report for 1998 (World Meteorological Organization, 1999).
6. SENSOR DEVELOPMENTS
NDBC has been collecting relative humidity measurements, which are archived as dew point from selected stations since 1989. Reliability has improved and post calibrations show that the measurements meet WMO accuracy standards (Breaker et al., 1998). Though these measurements are now recorded at about 20 stations, NDBC is waiting for the National Weather Service to make these measurements an official requirement before installing them on all stations.
NASA's Tropical Rainfall Measuring Mission has funded NDBC for rainfall measurements from several buoys. The measurements to date have been made with an optical rain gauge, were of poor quality, and were not archived. A siphon rain gauge, in use with some success in the TOGA TAO array, will be installed on these buoys this year.
REFERENCES
Anctil F., M.A. Donelan, G.Z. Foristall, K.E. Steele, and Y. Ouellet, 1993: Deep water field evaluation of the NDBC-SWADE 3-m discus directional buoy. J. Atmos. Oceanic Technol., 10, 97-112.
Breaker, L.C., D.B. Gilhousen, and L.D. Burroughs, 1998: Preliminary results from long-term measurements of atmospheric moisture in the marine boundary layer in the Gulf of Mexico. J. Atmos. Oceanic Technol., 15, 661-676.
Daniault, N., P. Blouch, and F.X. Fusey, 1984: The use of free-drifting meteorological buoys to study winds and surface currents. Deep-Sea Research, 32, 107-113.
Earle, M.D., K.E. Steele, and Y.L. Hsu, 1984: Wave spectra corrections for measurements with hull-fixed accelerometers. Proc. Oceans '84, Marine Technology Society, Washington, DC.
Earle, M.D. and L.E. Borgman, 1999: Statistical intercomparison of ocean wave parameters. Preprints Third Conf. on Coastal Atmos. and Oceanic Prediction and Processes, New Orleans, LA, Amer. Meteor. Soc. (in print).
Gilhousen, D.B., 1987: A field evaluation of NDBC moored buoy winds. J. Atmos. Oceanic Technol., 4, 94-104.
Gilhousen, D.B., 1988: Quality control of meteorological data from automated marine stations. Preprints Fourth Int. Conf. on Interactive Information and Processing Systems for Meteor., Oceanogr. and Hyrdrology, Anaheim, Amer. Meteor. Soc., 248-253.
Gilhousen, D.B., 1993: Recent field evaluations of a wind-measuring drifting buoy. Preprints Eighth Symp. on Meteor. Obs. and Instrum., Anaheim, Amer. Meteor. Soc., 304-310.
Hervey, R., 1999: Are moored buoy winds too low in high seas? Preprints Third Conf. on Coastal Atmos. and Oceanic Prediction and Processes, New Orleans, LA, Amer. Meteor. Soc. (in print).
World Meteorological Organization, 1999: Data Buoy Cooperation Panel Annual Report for 1998. DBCP Technical Document No. 13.
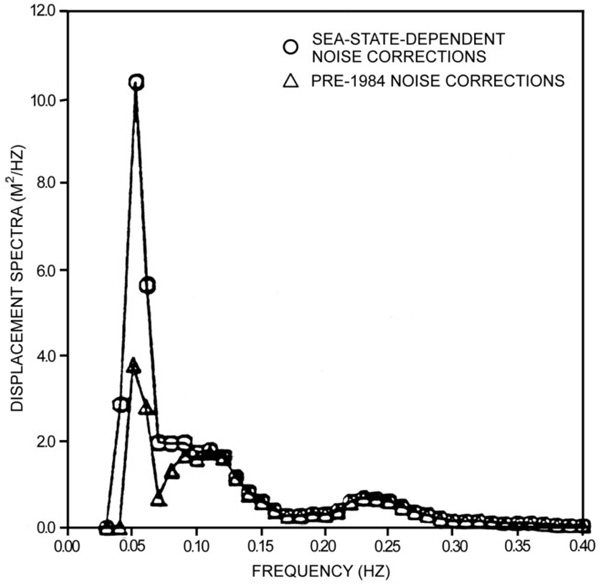